
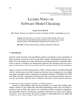
Recovering a physics-driven model, i.e. a governing set of equations of the underlying dynamical systems, from the real-world data has been of recent interest. Most existing methods either operate on simulation data with unrealistically high sampling rates or require explicit measurements of all system variables, which is not amenable in real-world deployments. Moreover, they assume the timestamps of external perturbations to the physical system are known a priori, without uncertainty, implicitly discounting any sensor time-synchronization or human reporting errors. In this paper, we propose a novel liquid time constant neural network (LTC-NN) based architecture to recover underlying model of physical dynamics from real-world data. The automatic differentiation property of LTC-NN nodes overcomes problems associated with low sampling rates, the input dependent time constant in the forward pass of the hidden layer of LTC-NN nodes creates a massive search space of implicit physical dynamics, the physics model solver based data reconstruction loss guides the search for the correct set of implicit dynamics, and the use of the dropout regularization in the dense layer ensures extraction of the sparsest model. Further, to account for the perturbation timing error, we utilize dense layer nodes to search through input shifts that results in the lowest reconstruction loss. Experiments on four benchmark dynamical systems, three with simulation data and one with the real-world data show that the LTC-NN architecture is more accurate in recovering implicit physics model coefficients than the state-of-the-art sparse model recovery approaches. We also introduce four additional case studies (total eight) on real-life medical examples in simulation and with real-world clinical data to show effectiveness of our approach in recovering underlying model in practice.