
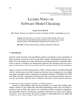
Optical microscopy is rapidly moving to the nanoscale to investigate biological specimens at molecular level using fluorescence as a mechanism of contrast (Diaspro A., Bianchini P., “Optical nanoscopy”, Riv. Nuovo Cimento, 43 (2020) 385). The latest results demonstrate the potential to provide information at the Angstrom level under standard conditions (Weber M., von der Emde H., Leutenegger M. et al., “MINSTED nanoscopy enters the Ångström localization range”, Nat. Biotechnol., 41 (2023) 569; Reinhardt S. C. M., Masullo L. A., Baudrexel I. et al., “Ångström-resolution fluorescence microscopy”, Nature, 617 (2023) 711). Other optical methods are growing to offer a kind of “tunability” in terms of spatial and temporal resolution to study the delicate and complex relationship between structure and function in cells (Diaspro A., Expedition into the Nanoworld. An Exciting Voyage from Optical Microscopy to Nanoscopy (Springer Nature) 2022). The modern optical microscope operates multimodally at the nanoscale using the decisive advantage provided by artificial intelligence algorithms (Pineda J., Midtvedt B., Bachimanchi H. et al., “Geometric deep learning reveals the spatiotemporal features of microscopic motion”, Nat. Mach. Intell., 5 (2023) 71).
New instruments, challenging theoretical approaches, and unique applications from the molecular level to humans are the link to discuss developments of optical microscopy in the realms of fluorescence, and label-free mechanisms of contrast, which are discussed in this volume.
The first contribution is by Colin J. R. Sheppard regarding image scanning microscopy, a technique of confocal microscopy in which the confocal pinhole is replaced by a detector array that allows a better signal-to-noise ratio. Here are discussed the developments and imaging properties of the image scanning microscope. Barbara Storti and Ranieri Bizzarri explain the relevance in the super-resolution domain of the main photophysical features of photochromic Förster Energy Transfer (pcFRET) and describe a powerful way to analyze data. The evolution of laser scanning microscopy approaches is documented by Giuseppe Vicidomini that introduces the fundamental impact provided by the possibility of performing single-photon detection, for example, by employing the asynchronous read-out available through the use of single-photon avalanche diodes. Luca Lanzanò and colleagues discuss how the analysis of fluorescence lifetimes can be boosted by the phasor analysis to perform super-resolution imaging. The separation of photons by lifetime tuning and the use of an image correlation spectroscopy approach are discussed as evolution of the stimulated emission depletion (STED) approach. The exploration of the eukaryotic nucleus by a multimodal single-molecule microscopy approach is a topic debated by Matteo Mazzocca and Davide Mazza. They critically discuss how to quantify nuclear proteins’ dynamics and interactions in living cells.
The tethered particle motion technique and magnetic tweezers are the topics of Laura Finzi and David Dunlap in the context of single-particle tracking and considering recent methodological advances and the application to the study of DNA supercoiling and transcription.
Brillouin scattering is related to a light-matter interaction that induces a frequency shift in the scattered photons. Antonio Fiore and Giuliano Scarcelli report utilizing this phenomenon as contrast mechanism in optical microscopy, enabling the label-free measurement of mechanical properties of cells, tissues, and biomaterials. Refractive index, speed of sound, and kinematic viscosity, as well as mass density and local absorption, are some of the biophysical applications of Brillouin microscopy. Laura Sironi and colleagues with Giuseppe Chirico contribute to laser microfabrication for optical sensing and imaging in vivo by providing an overview of the basics of photo-polymerization induced by two-photon excitation including two case studies focused on direct laser writing of biocompatible hydrogels and scaffolding for tissue and bone regeneration. Optical elements with rapidly adjustable diffractive properties are building blocks for advanced optical instruments, as discussed by Peter Saggau, who reports about applications in multimodal microscopy and nanoscopy.
The route to the Angstrom level performances of the fluorescence optical microscopes has its crucial role in the development of an original single-molecule localization scheme named MINFLUX, due to the minimal photon flux needed, that utilizes inhomogeneous and dynamic illumination to improve the position estimation precision, reaching isotropic, single-nanometer precisions. Francisco Balzarotti discusses this approach in terms of information theory in the scenario of single-molecule localization and imaging.
This volume is completed by the selected contribution of young researchers. Margherita Angelini and coworkers discuss using gold nanohole array plasmonic metasurfaces for sensing applications exploiting their optical properties and implementing a multi-signal platform. Payvand Arjmand and Marc Guillon debate some drawbacks in super-resolved microscopy related to the limit of penetration into the tissue, the acquisition speed, and the possible photo-bleaching. They address the use of compressed detection using the statistical properties of speckles, focusing on STED microscopy. Lisa Cuneo with Simone Civita and colleagues propose an artificial intelligence approach to separate the signal and background based on a scattering network approach coupled with a convolutional neural network (CNN), trained with images of background to which synthetic images of single molecules are added. The reduction of background is also the topic reported by Alessandro Passera and coworkers with Francisco Balzarotti. They show how to improve imaging speed using DNA-PAINT imaging and MINFLUX nanoscopy. Sabrina Zappone demonstrates fluorescence correlation spectroscopy to study how non-coding RNAs can play a role in alpha-synuclein phase transitions, taking advantage of a novel single-photon-avalanche-diode (SPAD) array detector.
Our bet is that, after reading this volume, you can return to your lab with at least a new idea.
P. Bianchini, A. Diaspro, C.J.R. Sheppard and M. Bouzin