
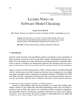
Coupled electron-nuclear spins are promising physical systems for quantum information processing: By combining the long coherence times of the nuclear spins with the ability to initialize, control, and measure the electron spin state, the favorable properties of each spin species are utilized. We present a vision of how these systems could be used as the fundamental processor of a quantum computer, in which the nuclear spins are analogous to local memory units, whereas the electron spins act as buses. In particular, we focus on control of a system in which a single electron spin is coupled to N nuclear spins via resolvable anisotropic hyperfine interactions. High-fidelity universal control of this 1-electron–N-nuclei system is possible exclusively using excitations on a single electron spin transition. This electron spin actuator control is implemented by using optimal control theory to find the modulation sequences that generate the desired unitary operations. A model to fully characterize decoherence of the nuclear qubits in this context is currently under investigation. Up to now, coherent control using an electron spin actuator in an ensemble of anisotropic hyperfine-coupled 1-electron–1-nucleus systems has been achieved, providing evidence that we can generate any unitary operation on such systems while sitting on a single transmitter frequency (Hodges J. et al., Phys. Rev. A, 78 (2008) 010303). Data was acquired using a custom-built pulsed electron spin resonance spectrometer. Complex modulation sequences found by the GRadient Ascent Pulse Engineering (GRAPE) algorithm (Khaneja N. et al., J. Magn. Res., 172 (2005) 296) were used to perform simple-preparation–quantum-operation–readout experiments. The next generation of the experimental setup will include an improved spectrometer, bandwidth-constrained GRAPE (Aiello C. and Hodges J., in preparation), and samples with larger Hilbert spaces.