
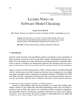
Several concepts have emerged related to endurance exercise performance and the first component issue is the level of aerobic metabolism that can be maintained during a race. The upper limit for this is maximal oxygen uptake (VO2max). This is usually achieved during relatively large muscle mass exercise and represents the integrative ability of the heart to generate a high cardiac output, total body haemoglobin, high muscle blood flow and muscle oxygen extraction. VO2 max is an important determinant of endurance performance which represents a true parametric measure of cardiorespiratory capacity for an individual at a given degree of fitness and oxygen availability. VO2 max values 50–100% greater than those seen in normally active healthy young subjects are seen in champion endurance athletes and the most adaptations to training that contribute to these high VO2 max values include increased cardiac stroke volume, increased blood volume, increased capillary density and mitochondrial density in the trained muscles. The primary distinguishing characteristic of elite endurance athletes that allows them to exercise fast over prolonged periods of time is a large, compliant heart with a compliant pericardium that can accommodate a lot of blood, very fast, to take maximal advantage of the Starling mechanism to generate a large stroke volume.
Why athletes stop exercising at VO2 max? Among some mechanisms presented in the literature, actual evidence show that severe functional alterations appear at the local muscle level due to what is ultimately a limitation in convective oxygen transport, which activates muscle afferents leading to cessation of central motor drive and voluntary effort.
Pulmonary oxygen uptake (VO2) measurements, though not without problems of data collection, analysis and interpretation, can provide valuable insights into metabolic control processes within exercising muscle. The primary component of VO2 kinetics gives a faithful representation of muscle VO2. One of the long-lasting debates in the field concerns whether or not the time constant of the primary component is slower for work rates above the lactate threshold (LT) compared to those below the LT. At these high work rates, a VO2 slow component manifests and is responsible for increasing the total metabolic O2 gain. The slow component phenomenon challenges some of the fundamental concepts in exercise physiology including the notion of steady-state VO2, O2 deficit, work efficiency and the control of muscle energetics. Mechanistic bases of this phenomenon are crucial to our understanding of muscle function and dysfunction. The VO2 slow component represents an excess VO2 that mandate an accelerated glycogenolysis which accelerate the fatigue processes. Slower VO2 kinetics obligate an increased O2 deficit and greater perturbation of the intracellular milieu that is accompanied by reduced exercise tolerance. Interventions that facilitate either a speeding of the VO2 response towards the expected steady state or a reduction in the magnitude of the VO2 slow component should therefore result in improved exercise tolerance. Augmented O2 delivery can speed the overall VO2 kinetics, increase the gain of the VO2 primary component and reduce the VO2 slow component. The most potent intervention is endurance exercise training. On a practical note, although a large number of studies have demonstrated that endurance training results in improved VO2 kinetics, the actual type (volume, intensity, frequency and duration) that optimise these effects remains to be elucidated.
It appears that the rate and magnitude of improvement in VO2 max and VO2 kinetics with training may be dissociated, suggesting different mechanisms.