
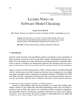
The reversible aggregation of human red blood cells (RBC) continues to be of interest in the field of hemorheology [1-12], in that RBC aggregation is a major determinant of the in vitro rheological properties of blood. In addition, the in vivo flow dynamics and flow resistance of blood are influenced by RBC aggregation [13]. Measures of RBC aggregation, such as the erythrocyte sedimentation rate (ESR), are commonly used as diagnostic tests and as one index to the efficacy of therapy (e.g., following drug therapy in rheumatoid arthritis); in diabetes mellitus RBC aggregation is normalized by improved glycemic control [14] There is now general agreement regarding the correlations between elevated levels of fibrinogen or other large plasma proteins and enhanced RBC aggregation, and the effects of molecular mass and concentration for neutral polymers such as dextran [15]. However, the specific mechanisms involved in RBC aggregation have not yet been elucidated, and thus it is not yet possible to fully understand the relations between pathology and altered RBC aggregation.
RBC form multi-cell linear or branched aggregates in vitro when they are suspended in either plasma or solutions containing large polymers (e.g., dextran ≥ 40 kDa); the linear forms are often termed rouleaux since they resemble a stack of coins. In vivo RBC aggregation occurs at low shear forces or stasis and is a major determinant of low shear blood viscosity and thus in vivo flow dynamics [13]. It is important to note that RBC aggregation is a reversible process, with aggregates dispersed by mechanical or fluid flow forces, and then reforming when the forces are removed. Conversely, RBC agglutination and blood coagulation are irreversible processes due to either protein polymerization or strong antigen-antibody attractive forces. Abnormal increases of RBC aggregation have been observed in several diseases associated with vascular disorders (e.g., diabetes mellitus or hypertension). RBC aggregation is primarily determined by RBC aggregability (i.e., the intrinsic cell characteristics affecting RBC aggregation) and by the concentration of the inducing macromolecule or the plasma level of large proteins [16]. In blood, fibrinogen is one of the most important determinants of blood viscosity due to its strong tendency to increase both plasma viscosity and RBC aggregation [17]. In the past, most reports dealt primarily with the ability of plasma proteins to promote aggregation; for example higher fibrinogen levels have been linked to elevated blood viscosities in hypertensive patients [18].
At present, there are two co-existing models for RBC aggregation: bridging and depletion. In the bridging model, red cell aggregation is proposed to occur when the bridging forces due to the adsorption of macromolecules onto adjacent cell surfaces exceed disaggregating forces due to electrostatic repulsion, membrane strain and mechanical shearing [15, 19-23]. This model seems to be similar to other cell interactions like agglutination, with the only difference being that the proposed adsorption energy of the macromolecules is much smaller in order to be consistent with the relative weakness of these forces. In contrast, the depletion model proposes quite the opposite. In this model RBC aggregation occurs as a result of a lower localized protein or polymer concentration near the cell surface compared to the suspending medium (i.e., relative depletion near the cell surface). This exclusion of macromolecules near the cell surface leads to an osmotic gradient and thus depletion interaction [24]. As with the bridging model, disaggregation forces are electrostatic repulsion, membrane strain and mechanical shearing. Several previous reports have dealt with the experimental and theoretical aspects of depletion aggregation, often termed depletion flocculation, as applied to the general field of colloid chemistry [25-28]. However, polymer depletion as a mechanism for red blood cell aggregation has received much less attention, with only a few literature reports relevant to this approach [24, 29-33].