
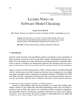
The goal of this book is to improve understanding of what is meant by the terms “systolic function” and “diastolic function” and how these can best be measured and interpreted, both now and in the future..
In Diastole, the ventricular wall must be appropriately compliant to allow rapid, complete filling at low filling pressures. The key questions are:
• What structures and mechanisms determine ventricular compliance? Does compliance primarily reflect the myocytes (titin, tubulin, other elastic intramyocytic proteins)? The collagen extracellular matrix? Pressures, distentions, and flows in fluid compartments (blood, lymph, interstitial fluids)? What is the role of calcium-dependent myofilament interactions (and incomplete relaxation)? Is compliance subject to endothelial modulation? Is it viscoelastic?
• Is elastic systolic energy normally stored in the heart wall to aid filling during diastole (ventricular suction)? If so, where is this energy stored ... in myocytes? in collagen? In what mode and direction ... compression? expansion? torsional deformation, twist, or other shears? Does the contractile state of the myocytes during systole alter diastolic suction? How is such energy storage transmurally distributed? Is it greater nearer the epicardium? the endocardium? uniform throughout the wall? What is the timing of elastic energy release during the cardiac cycle? Is all of the potential energy stored during systole dissipated during relaxation? Is suction normally in evidence or is it a backup mechanism? How does one demonstrate when suction is present? Does energy storage in the mitral valvular-ventricular complex contribute to filling?
• What is the effect of overall chamber geometry (size, shape) on compliance and suction? What are the effects of geometric remodeling (protein turnover with widely different time courses), heart rate, pericardial constraint, ventricular interdependence, asynchrony and asynergy, exercise, hypertrophy, dilatation, heart failure? What is the relationship between diastolic chamber properties and myocardial (muscle) properties?
• Can diastolic performance be measured adequately in the clinical setting and are the measurements clinically meaningful? How important is rapid early diastolic filling? How are regional pressure gradients produced within the ventricle? How should one define ventricular volume in chambers which have valve leaflets opening, closing and deforming? How should relaxation be defined? What are the effects of various clinical interventions and surgical procedures on diastolic performance?
In Systole, the ca. 109 myocardial cells in the ventricular wall must generate adequate coordinated force to provide sufficient pressure to open the outlet valve and then shorten and thicken the wall to pump an appropriate stroke volume. The key questions addressed are:
• Because measured wall thickness increases may not be accounted for solely by cell thickness increase and wall curvature changes, what cellular rearrangements are involved? Fiber slippage? Fiber direction changes? Do sheets of quasiradially oriented fibers contribute to shear-induced wall thickness increase? Do additional fibers run at steep pitch angles from epicardium to endocardium? Does the spiral architecture of the cells lead to systolic torsional deformations which: (1) allow the myocardium to thicken as much as it does within the constraints of sarcomere shortening; and (2) distribute the work of the heart uniformly from endocardium to epicardium by equalizing transmural differences in fiber shortening? Does epicardial shortening “crush” and rearrange the endocardial fibers such that endocardial cross-fiber shortening greatly exceeds fiber shortening?
• How are myocardial cells tethered to one another? Is myocardium stiffer in the fiber direction than in the cross-fiber direction? What are the effects of the spiral-wrapped ventricular wall on transmural dynamics? Why is torsional deformation coupled tightly to chamber volume during systole, but almost uncoupled in diastole? How much regional variation is observed in contractile parameters? Does the volume of the tissue change during contraction? How does the endocardial endothelium affect force generation?
• Can we deduce myocyte contractile properties from measurements at the chamber level (e.g., pressure, volume, torsion) or isolated muscle level (e.g., force, length)? Can we deduce cellular energetics (e.g., oxygen, ATP consumption) from measurements at the chamber level (e.g., pressure-volume area)? How does the force-interval relationship (mechanical restitution curve) relate to calcium kinetics of various cellular compartments?
• How important is the quest for a single index of ventricular “contractility.” How do the concepts of maximal elastance, preload recruitable stroke work, dP/dt, dP/dtmax vs. EDV slope, and maximal power compare in this regard? Does valvular elasticity confound the interpretation of these indices? Is the concept of ‘contractility’ clinically useful? Does an increase in perfusion increase contractility and oxygen consumption?
• How can we define the load on the left ventricle? Is it wall stress? Chamber pressure? Arterial impedance? Various combinations of these, depending on the application?
• Is adrenergic regulation of the force-frequency relation a major determinant of intrinsic contractility in the intact heart (in addition to length-dependent activation, direct beta adrenergic stimulation of the myocardium, and the basal force- frequency relation)? If so, how is it achieved?
• What are the effects of pathophysiological conditions (infarction, hypertrophy, dilatation, stunning, hybernation) on systolic performance?
In a recent editorial [Circulation 91: 1901, 1995], Claude L’ enfant predicted an important role for integrative physiology to help make sense of the important bits and pieces of new knowledge that are so rapidly accumulating from subcellular and molecular biology. He concluded that “...the full realization of the implications of this vi new knowledge will not occur without the concurrent and inspired efforts of scientists who apply this information to the larger organism and use it in the context of the vast knowledge already assembled about living creatures.” It is hoped that the questions addressed in this volume present the most recent views of integrative cardiovascular physiology and focus on the vital issues in this cooperative effort.
Neil B. Ingels, Jr.
George T Daughters
Jan Baan
James W. Covell
Robert S. Reneman
Frank C-P. Yin